A small nuclear reactor with a difference
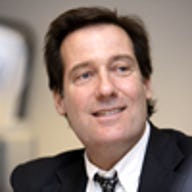

But for that to truly happen, development of what the industry likes to call “small modular reactors” (SMR) will have to take a decided turn toward alternative technologies that depart from conventional reactor designs in aspects other than size. In the U.S., recent SMR activity has focused on what are essentially shrunken versions of traditional reactors that use solid uranium fuel rods housed in a traditional cladding, and that are cooled by normal water, known in nuclear parlance as "light water." The heat picked up by the coolant in these "light water reactors" (LWRs) turns to steam that drives a turbine to generate electricity.
While NuScale and B&W have taken important steps by impressively engineering mini-reactors that in principle will deliver affordable and safer than ever nuclear power, the real game changer will come with the advent of altogether different reactor technologies that operate far more efficiently than conventional reactors designs and thus enhance the cost advantage and make nuclear more competitive with rival energy forms like cheap natural gas.
These new reactors will also minimize nuclear waste and weapons proliferation threats. And in departing from the uranium fueled, water-cooled design that has defined the industry for its 50+ years, they portend safer and higher-temperature operations that can serve not only as efficient electricity generators but also as sources of clean industrial process heat, replacing today's fossil fuel furnaces, as U.S. Energy Secretary Ernest Moniz notes.
In fact, “small modular reactor” and “alternative nuclear technologies” go hand-in-glove, as many of the unconventional designs are well-suited to small sizes. I've written about several of them here on SmartPlanet, including molten salt reactors from companies including Terrestrial Energy, Thorium Tech Solution and Transatomic Power, and pebble bed reactors from the likes of Steenkampskraal Thorium Ltd., X-Energy and Northern Nuclear.
“Our innovation is focused on price,” says Robert Schleicher, a General Atomics senior scientist and the co-inventor of EM² who I met on a recent tour of GA's development facilities in San Diego. “Can you get the cost down? That's the only way you can play in the world - by innovating new materials, getting the efficiency way up, simplifying the design and getting the cost into the competitive range.”
GA says that EM² will convert heat into electricity at nearly twice the efficiency of a conventional reactor – 53 percent versus what it says is somewhere between 28 percent and 34 percent for today's large commercial reactors. Each point of efficiency saving translates into a significant reduction in operating costs. Whereas today's large reactors produce electricity at 11 cents (U.S.) per kilowatt hour, the EM² will deliver at 6.5 cents, GA says.
SCORING POINTS
GA's design calls for a 265-megawatt (electric) sized reactor, with a fuel cycle lifetime of 30-plus years. Users could install as few or as many modules as their budget and requirements dictate. A small or low-budget user might want only one; a utility might start with one and add on others later. If a utility were to install 4 or 5 reactors, it would have over a gigawatt of capacity, roughly comparable to a conventional LWR, all on a little over 20 acres (for 4 units), or about a quarter of the footprint of a standard LWR according to GA.
“In our baseline plant, a four-module plant, one point of efficiency is worth a billion dollars in revenue over the life of the plant,” says Schleicher. “So those points are really important.” Using the highest estimated conventional efficiency of 34 percent, a utility would find an extra $19 billion in its pocket, or $25 billion when compared to a 28 percent efficient LWR.
The reactor is meant to operate at around 850 degrees C compared to 320 degrees C for an average LWR. To accommodate that heat, GA is developing a silicon carbide ceramic cladding that should be far more tolerant of high temperatures than the metallic cladding -- typically zircaloy -- used in LWR fuel rods and which would melt down at EM2 temperatures.
TAKING THE HEAT
“It's kind of our 'miracle material,'” says Schleicher. “This cladding doesn't melt.” According to Schleicher, the material would remain intact at up to well over 2,000 degrees C in the event of an emergency. “So you have this huge headroom between the operating temperature and what would ultimately fail,” he notes. “You could tolerate a huge number of adverse events and be safe.”
General Atomics recently completed 9 months of successful, rudimentary testing of the material in Tennessee at Oak Ridge National Laboratory's High Flux Isotope Reactor (HFIR), a test reactor that has a high neutron flux and can emulate the “fast spectrum” that EM² will deploy (EM² will not slow down neutrons the way today's “moderated” reactors do). This neutron bombardment not only helps use the fuel more efficiently, but also requires a novel cladding such as GA's silicon carbide that will stand up to it.
If the ceramic cladding performs as expected, it should answer the U.S. Department of Energy call for “accident tolerant” fuels, issued following the March 2011 meltdowns and explosions at Japan's Fukushima Daiichi reactors. Not only will the new silicon carbide cladding hold up to adverse conditions, but its ceramic nature, in combination with the helium coolant, virtually eliminates the possibility of a hydrogen explosion. The Fukushima reactors blew up from the build up of hydrogen from the metallic fuel cladding which was in contact with corrosive steam and water; the chemical natures of silicon carbide and helium would all but rule out any chance of a Fukushima-like hydrogen flare.
WASTE NOT
As for the fuel itself, GA has engineered another impressive advance: The cladding will surround cylindrical fuel rods that the company says will burn for 30 years, using a mix of depleted uranium (what's left over after processors enrich natural uranium for use in conventional reactors) and spent fuel – of which there is plenty already available in the form of "waste" from the world's LWRs.. After 30 years, GA would remove the fuel and separate out short lived fission product waste from the mix of left over heavy metals -- like plutonium -- which would all remain together and would not be removed individually, rendering them ineffective for any weapons use.
While the innovative high temperature fuel/cladding combination will be one of the keys to the 53 percent efficiency that will cut operating costs nearly in half, it won't be the only one. So too, will the helium coolant – helium can transfer heat at much higher temperatures than the light water that traditional reactors use.
And then there's GA's method for transferring the helium's heat into a turbine: It has engineered a Brayton cycle method that will funnel the helium straight into the turbine without first transferring it and less efficiently converting it to steam, the way conventional reactors do with Rankine cycle turbines. That method will deliver a 48 percent efficient heat-to-electricity conversion rate. GA will then boost the overall number to 53 percent by adding a Rankine cycle that captures otherwise lost heat.
EM², which is designed to sit underground for extra security against potential attack, comes with other innovations as well: Its above ground housing will include ribbed outer walls designed to repel or shred any aircraft that might intentionally fly into it.
It will also rely on air for any unlikely need of emergency cooling. This passive cooling system, known as a Direct Reactor Air Cooling System (DRACS), would not require power. Passive cooling is becoming more common even in modern versions of conventional reactors; the failure of auxiliary generators at Fukushima's “actively” cooled reactors factored into the meltdowns there. The company vied unsuccessfully for SMR funding in the two DOE rounds awarded to B&W and NuScale.
GA believes it can build a commercial model within 12 years for around $4 billion. That timeline would include a completed prototype after 10 years.
TEAMING UP
It is searching for partners in industry and in government both domestically and abroad to help it develop, build and finance EM². GA is working with nuclear engineering companies Chicago Bridge & Iron (through CB&I's acquisition of the Shaw Group) and Mitsubishi Heavy Industries; government supporters include the U.S. state of Mississippi.
CB&I has experience with, among other nuclear project, SMRs – Shaw helped Westinghouse to develop a conventional SMR. Westinghouse, like GA, failed to secure DOE SMR funding. Following the government's recent award to NuScale, Westinghouse announced it is backing off SMRs. GA vied unsuccessfully for SMR funding in the second DOE round that was awarded to NuScale.
Westinghouse is not the only conventional SMR developer that is showing signs of distress. Babcock & Wilcox, which won a DOE pledge for up to $225 million in late 2012, earlier this year said it might make considerable cuts to development because it has been unable to find a financial partner – the company had been seeking to sell 70 percent of Generation mPower, its SMR joint venture.
Perhaps the moral in the rough and tumble SMR story at Westinghouse and B&W is: Don't just go small. Go different. It's a lesson that GA has already learned and is trying to put into practice.
Photos of Robert Schleicher, Christina Back are by Mark Halper. Mock-ups are from General Atomics.- Could Ukraine crisis spur new forms of nuclear energy?
- Emergency pollution measures: China steps up development of thorium nuclear
- Senate bill would slash U.S. reliance on China for vital metals while also securing an energy future
- Business! Innovation! Startups! It must be nuclear power
- Bombs away: Key uranium supply to U.S., from Russian weapons, ends. Time for thorium?
- U.S. Energy Secretary: Use nuclear reactors for clean industrial heat
- Novel nuclear reactors atop MIT contest finalists
This post was originally published on Smartplanet.com