Bioprinting bones and muscles: The inkjet cell printers shaping the future of transplants
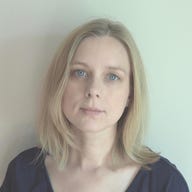

An ear and jaw bone piece printed by WFIRM's bioprinter.
14 years ago, most businesses hadn't even heard of 3D printing, let alone experimented with printing objects in material like plastic or metal. But one research institute was already laying the foundation for building its own 3D printer for an altogether more complex material: human tissue.
Tissue and organs transplants have been used in medicine for decades to help patients whose own tissue has become diseased or damaged -- skin grafts for burns victims, for example, or using a piece of patellar tendon to replace a ruptured ligament. Typically they come from donors or are moved from a healthy part of a patient's body to a damaged part, but scientists from Wake Forest Institute for Regenerative Medicine (WFIRM) have developed a prototype printer that could one day be used to print tissue sections designed to fit a person's unique condition.
Initially, WFIRM researchers began working on building human tissue from cells by hand. First cells were removed from patients through biopsies -- where small samples of tissue are explanted to help physicians with diagnoses -- then cultured, to increase numbers. The cells are put on a scaffold, placed into an incubator and, once the tissue has grown into the desired construct, returned to a patient. It was a labour intensive process, and one that yielded tissue that wasn't strong enough to be used in human patients.
"We had been working on tissues and organs for patients for implantation and we had already put a couple in patients. We realised it was OK to create these by hand, if you were creating a few for clinical trials, but if you were creating it for hundreds of thousands of patients, you would need to scale it up. That's when we started looking at how we could scale up the technology," Dr Anthony Atala, who heads up the research at WFIRM, told ZDNet.
The ITOP system at work.
The WFIRM researchers hit on the idea of using printers to make tissue a few years later, but with commercial 3D printing still very much in its infancy, the researchers were experimenting with more widespread technology: adapting existing desktop inkjet printers.
The cells were put into the printers' inkwells, and then outputted in a particular way to create the desired tissue construct.
While commercial 3D printers became more widespread over the years that followed, WFRIM researchers had to develop their own hardware based on the inket system due to the unique nature of human tissue.
"We started using the inkjet printer to experiment, to see how we could make it work... we could get the cells through the hydrogel, but we couldn't get the precision in terms of where we laid the cells down, and the structures didn't have the structural integrity necessary to be implanted surgically. We started looking at more sophisticated printing that we could achieve, and we started doing that by basically constructing our own printing devices," Atala said.
For example, the WFIRM system, known as Integrated Tissue and Organ Printing System (ITOP), needed to be of incredibly high precision, able to print out structures with accuracy of around two microns -- an eightieth the size of a human hair.
In addition, while many 3D printed materials can be extrude directly, the cells are outputted in gels to protect them. "It's very important to make sure you lay down the cells without damaging them. It's critical, because if you start out with dead cells before you even finish your product it's not going to work," Atala said.
Download this ebook
In order to build a tissue of the right size and shape, the area where the structure will go is scanned in three dimensions -- using traditional medical techniques like CT and MRI scanning -- and the WFIRM's printer creates a digital construct that can then be printed for real.
The system not only prints the hydrogels that contain the cells, it also prints out the structure made of what WFIRM describes as "plastic like materials" that is used to give the tissue its shape.
"We also put together a system of bioinks that give it strength and structural integrity -- creating bioinks that are soft, hard, soft, hard and that re-interconnected," Atala said. The alternating soft and hard materials are laid down similar to building with Lincoln Logs, Atala said, making the structure tough enough to withstand being implanted surgically.
The structures are also built to include tiny microchannels, to allow nutrients to flow into the new tissue. While in the past, creating tissue without any blood vessels already built in meant that constructs could only be a very small size -- 200 microns or 0.007 inches. Over that size, and nutrients can't get to all the cells in the tissue, a problem that Atala and his team have circumvented by adding the microchannels.
Atala's team has made a tissue structure -- a tiny version of a human ear -- around 1.5 inches in size. The structure showed signs of vascularisation in its outer regions one and two months after implantation -- a pattern similar to that seen in native cartilage. That means that the body that tissue implanted had begun to treat the tissue as its own, growing the blood vessels the tissue would need to survive and integrate into the patient long term.
So far, the researchers have 3D printed sections of muscle, cartilage, and bone -- tissues with very different strengths -- and are now undertaking the preclinical studies necessary before the printed tissue can be put into human patients.
The uses for the technology are many: bioprinting skin onto burns, or onto directly wounds at the site of injury. Potentially, the ITOP system could even create partial organs for implantation in human patients.
"With this, we can now create tissue structures that are human scale," Atala said.
"In terms of engineering, we've been able to create flat structures such as skin and they're the least complex. Tubular structures are the second level of complexity, like blood vessels. Hollow non-tubular organs like the stomach or the bladder are the third level of complexity and solid organs are the most complex - the heart, the liver, the lung,. That's the going to be the same for the printing, it's going to the be the same sort of strategy from least complex to most complex in terms of achieving what we need for the future."