Pencil + sticky tape = desktop supercollider + post-silicon processors
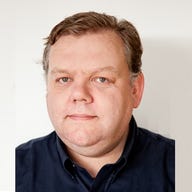
It is in the nature of physics that some of the weirdest things live in the plainest view. When you were a kid on your summer holidays, a day at the beach meant a dip in the sea and sandcastles on the beach: what's not to understand? Yet water is an extremely strange liquid compared to any remotely similar compound: it gets bigger when it freezes; when it does freeze, melts or vapourise it does so at all the wrong temperatures; and it is a remarkable solvent. Sand is half silicon, something that's rubbish at being a metal and not very good at being a insulator but absolutely superb at giving us control over electricity.
Now it looks as if the pencils you kept in your school pencil case contain even greater mysteries – and huge potential. It's long been known that carbon has two stable forms, diamond and bulk carbon like the graphite in a pencil lead. As the basic mechanisms of atomic bonding were unravelled in the last century, it became clear that there were other theoretical states based on carbon's ability to form a a hexagonal honeycomb structure. By the 1980s, some examples had been found – buckyballs, where the honeycomb wraps to form a sphere, and nanotubes, where it curls into a long tube. The most interesting variant, graphene, was not to be found.
Graphene is made from stacks of very flat sheets of hexagonally linked carbon atoms. The atomic bonding between sheets is relatively weak compared to that between the atoms in each sheet, meaning it's easy for sheets to slip apart – which is why pencils leave such excellent skidmarks on paper. If it were possible to produce single sheets of graphene, quantum physicists mused, it would have even more remarkable properties: electrons would effectively lose their mass and move more like waves through free space, not particles through a lattice. (Electrons, of course, are neither waves nor particles although they have wave and particle nature. Different circumstances let them behave in different ways). But there was a general consensus that a single sheet of graphene would be so vulnerable to environmental disruption that these thoughts would remain theoretical.
Until 2004, that was that. Then, by the insanely simple expedient of using adhesive tape to peel off layers from ordinary graphite, then rubbing those layers gently against an oxidised silicon surface, UK researcher Andre Geim from the University of Manchester's Mesoscopic Physics Group not only produced atom-thick graphene sheets but proceeded to show both that they're perfectly stable in a normal environment and that the stuff does indeed have a weirdness coefficient approaching infinity. (Geim also levitates frogs and steals intellectual property from geckos – he's one to watch).
With ordinary conductors, electrons move quite slowly through the crystal lattice. They bump into atoms and get deflected, lose energy, do all the sorts of things that make electronics get warm and batteries go flat. The unique configuration of graphene means that none of the above happens: the forces that an electron experiences in there means that it just doesn't see any obstacles. It stops interacting with its surroundings, inside or out of the graphene sheet, and starts to behave like a massless object travelling near to the speed of light. In fact, it looks remarkably like a neutrino – but one with an electric charge.
This has some startling consequences. Graphene is abnormally conductive, with about half the resistivity of copper, and is able to support a much higher current density. Because electrons move so fast within it with such low loss – behaviour known as ballistic conductivity -- it should be able to produce a device called a ballistic transistor, with extremely high switching speeds and efficient operation. In fact, graphene is already being talked about as one of the better candidates to take up the flame of Moore's Law if silicon runs out of puff as expected sometime in the next decade.
These thoughts are fuelled not only by the substance's unique way with electrons, and the way that quite gross irregularities and lacunae within the lattice have little effect on its behaviour, but because it should lend itself to mass-production techniques far easier than its close cousin and fellow Moore's Law contender, the carbon nanotube. There's talk of using nano-stencils to build entire processors out of sheets of graphene cut into active devices and waveguides: well, perhaps. Single-sheet graphene is just two years old; its physics is poorly understood and there's very little literature on it, even if that hasn't stopped IBM producing a graphene transistor in its labs that demonstrates many essential features.
But still, if that was all graphene promised, it would be exciting enough. All of the above, though, is barely scratching the surface.
Remember that an electron, normally a particle with mass and a tendency to hit things, behaves like a neutrino within graphene. It has effectively no mass and travels at just about the speed of light. Yet that speed of light is not c, the speed of light in a vacuum: graphene's electronic speed of light is some 300 times slower.
But because exactly the same equations and behaviour apply to graphene's ballistic electrons at their speed of light as do to normal particles at c, all sorts of real-world physics becomes open to exploration. Classes of unfeasibly energetic experiments which rely on making ordinary particles go at close to c become entirely possible with graphene electrons and far more modest resources.
Geim and his co-workers suggest one such. Normal electrons can tunnel through potential barriers: as the barriers get higher the tunnelling becomes exponentially less likely to happen. Yet theory suggests that at very high speeds and with very high barriers, electrons will start to find it easier to get across as the barriers get taller. This is called the Klein Paradox, and demonstrating it in the universe outside graphene would need the sort of conditions you find close to an exotically heavy nucleus or, if you're particularly keen, a black hole. In graphene, you may be able to recreate an appropriate barrier with an ordinary semiconductor junction: there's talk of desktop super-colliders.
Other areas that graphene may illuminate include direct observation for the first time of relativistic electrons generating their own anti-particles – and there are even suggestions that some aspects of string theory, long thought to lie well beyond practical observation, may become testable.
All these thoughts has sparked a rush to explore graphene: new ideas are appearing just about as fast as people can think them up. While the ultimate impact of these discoveries will take many years to work out, few in the field deny that a a new class of physics, with considerable practical and theoretical implications, is opening up. Not bad for a bit of pencil lead and some sticky tape.