Supercomputers help design better golf balls
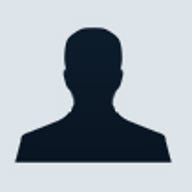
Researchers are using supercomputers to create new balls that will improve the game of avid golfers by flying farther. They've used the supercomputers at Arizona State University to simulate the physics of golf balls and to model how air flows around a ball in flight and to study how this flow is influenced by the ball's dimples. Their goal is to make a better golf ball by optimizing the size and pattern of these dimples and lowering the drag golf balls encounter as they fly through the air. 'For a golf ball, drag reduction means that the ball flies farther,' says one of the engineers. A single simulation takes about 300 hours by using 500 processors -- so these balls could be expensive to produce. And the scientists are the first to admit that new golf balls based on their research are years away. But read more...
You can see above the results of a "direct numerical simulation of the flow around a dimpled sphere at Re=110000. (a) Side view of the wake visualized by spanwise vorticity isolines.; (b) Detail of the mesh in a dimple; (c) Visualization of the shear layers in a single dimple. The area shown is the one indicate my the circle in part (a) of the figure." (Credit: Elias Balaras group, University of Maryland). Here are two links to a larger version of this figure and to Balaras's research gallery. Please note that in the above caption, Re is an abbreviation for the Reynolds number. (Credit: Wikipedia) And here is an additional link from where you'll be able to watch several movies about these simulations.
This research work has been conducted by a team of five researchers. Clinton Smith is a Ph.D. student working at Arizona State University (ASU) with Kyle D. Squires, Professor of Mechanical Engineering at ASU. The two researchers have collaborated with Nikolaos Beratlis, Graduate Research Assistant in the Fischell Department of Bioengineering at the University of Maryland led by Elias Balaras, Associate Professor of Bioengineering, and with Masaya Tsunoda of Sumitomo Rubber Industries, Ltd.
Now, let's look at how dimples can improve the flight of a golf ball. "Once in flight, a golf ball experiences aerodynamic forces generated from the surrounding air flow as well as gravity. The latter constantly pulls it towards the ground, while the aerodynamic force in the direction of motion, or drag force, dictates the distance it travels. The main purpose of dimples is to reduce the drag and help the ball fly farther. Actually, dimpled golf balls experience about half the drag as those with no dimples. Although the United States Golf Association (USGA) regulates the design of golfballs, laying out uniform size and weight specifications that all approved golf balls must meet, the dimple pattern is not regulated. It is one of the very few parts of the ball over which companies have freedom to change the design."
Of course, the question -- difficult to answer -- is: "what pattern is best for lowering the drag? Up to now, dimple design has been more of an art than a science. For many years, sporting goods companies would design their dimple patterns by simple trial and error, testing prototype after prototype against one another. The new study takes a different approach, asking how to design dimple size and pattern based on mathematical equations that model the physics of a golf ball in flight. Working out the solution to these equations -- even on the fastest personal computers today -- is not feasible since it would take more than 15 years of computing time just to get a glimpse of the flow around the golf ball for a fraction of a second."
This research work has been presented on November 23, 2008 at the 61st Annual Meeting of the American Physical Society's Division of Fluid Dynamics under the title "Direct Numerical Simulations of the Flow around a Golf Ball: Effect of Rotation." Here is a link to the abstract. "Golf ball flight is affected by rotation of the ball (lift generation) and dimpling on the surface (drag reduction). Direct Numerical Simulation (DNS) is being developed for the flow around a rotating golf ball using an immersed boundary method. Adding to the computational cost is that the moving body must be re-located as the ball rotates. In the present effort, interface-tracking of the moving body is optimized using the Approximate Nearest Neighbor (ANN) approach. The code is parallelized using domain decomposition and message passing interface (MPI), and parallel performance results are presented for a range of grid sizes. Results are presented from a series of validation cases for flow over a smooth sphere and a golf ball."
This research work is also available from arXiv.org under the title "Visualization of flow over a golf ball at Re = 110,000." (November 5, 2008). Here is the beginning of the abstract. "The drag on a golf ball can be reduced by dimpling the surface. There have been few studies, primarily experimental, that provide quantitative information on the details of the drag reduction mechanisms. To illuminate the underlying mechanisms, Direct Numerical Simulation (DNS) is applied to the flow around a golf ball using an immersed boundary method. Computations are performed using up to 500 processors on a range of mesh resolutions from 61 million points to 1.2 billion points. Results are presented from simulations performed at a Reynolds number of Re = 110,000 using a grid of 1.2 billion points." Here is a link to the paper (PDF format, 2 pages, 58 KB).
For more information, you also might want to read previous papers. Here are some references I recommend.
- Dimples and drag: Experimental demonstration of the aerodynamics of golf balls (American Journal of Physics, Volume 75, Issue 8, Pages 764-767, August 2007)
- Taking flight on the course (ASU Research Magazine, March 2007)
- A Better Golf Game (American Institute of Physics, January 1, 2005)
Sources: American Institute of Physics (AIP) news release, November 24, 2008; and various websites
You'll find related stories by following the links below.