Throwing rocks at CO2

See update added at the bottom of the page.
Last week's column discussed research into the development of materials that could pull carbon dioxide out of the open air, and their limitations in helping to avert global warming. The compounds being developed in those projects were typically inexpensive polymers. Some readers, most notably the science journalist Elmar Veerman of the Netherlands, subsequently reminded me of a different approach to removing atmospheric carbon dioxide that I had not discussed -- one that involved completely natural materials and emulated the planet's own processes for removing the gas from the air. The idea deserves some follow-up discussion of its own.
Carbon dioxide (CO2) in the air doesn't remain there indefinitely. Green plants absorb a certain amount of it as they grow, converting it into cellulose and other carbohydrates through photosynthesis. Over a period of decades or centuries, however, CO2 is also taken up by rocks as it spontaneously reacts with silicate minerals. This reaction, which geochemists call mineral carbonation, is part of the routine weathering process, and it annually binds up roughly 100 million tons of carbon that way. Over vast geological expanses of time, those weathered rocks are drawn deep into the earth at subduction zones, allowing the CO2 to one day be released again during volcanic eruptions.
Artificial weathering
In a 1990 letter to the journal Nature, W. Seifritz of Windisch, Switzerland, suggested that silicate minerals might offer an easy way of disposing of CO2 and reducing its greenhouse effects. The silicate mineral olivine, for example, is the major constituent of the earth's upper mantle, and it is abundant in many rock formations at or near the surface. In the presence of water, the magnesium atoms in olivine combine readily with CO2 to form molecules of magnesite, whereas the silicon and oxygen rearrange into silicate quartz.
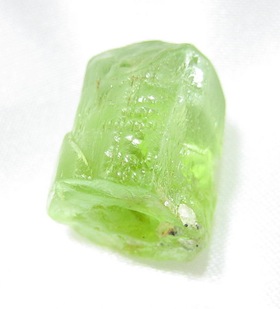
Seifritz envisioned pumping CO2 into watery suspensions of pulverized silicates to bind it, then flushing the products into the deep ocean. His rough sketch of an idea inspired investigations into the use of olivine to treat the flue gases from coal-burning power plants and other big CO2 emitters. Quarried olivine could be transported to those sites, ground into a powder, then mixed with streams of purified CO2 from the plants' smokestacks to absorb it. Some engineers are still trying to develop this approach, but it tends to be expensive: getting the olivine, capturing and purifying the CO2 from the plant smokestacks, and hauling away the weathered waste minerals and water takes a lot of money and energy.
Another mineral carbonation idea under study involves leaving the olivine in the ground and instead bringing the CO2 to it. Liquefied CO2 from industrial plants can be injected thousands of feet deep underground into cracks and microscopic holes in formations of basalt. Because the basalt is dense and nonporous, the CO2 should not be able to percolate back to the surface, and small amounts of olivine in the basalt should be able to absorb the CO2 within a few decades. This technique would therefore tie up the CO2 more securely than conventional sequestration strategies that involve storing it as a gas or liquid inside abandoned oilfields and similar formations, which might be appealing with respect to environmental safety. A pilot study of this approach is being planned by Battelle and the Pacific Northwest National Laboratory is now underway in for Wallula, Wash., where researchers seek to inject 1,000 tons of CO2 were injected into a 4,000-foot-deep well. [Note: Thanks to Annie M. Haas of Pacific Northwest National Laboratory for that correction; a timeline for the project is still under development.]
One potential limitation, however, is that as the rocks take up carbon dioxide, however, their surfaces may build up a so-called passivating layer of silica that could slow or block further uptake of the gas. How much that might undercut the success of the approach needs to be determined. And of course, this approach, too, suffers from the big standard sequestration expenses of capturing the CO2.
Scattered to the winds
A far simpler but more audacious solution is favored by R.D. "Olaf" Schuiling, a professor of earth sciences and emeritus chair of geochemistry at Utrecht University, and the Dutch Olivine Foundation for CO2 Reduction. At the foundation's SmartStones website, Schuiling advocates for mining olivine, grinding it to a fine powder, then spreading the dust throughout humid tropical areas. Doing so, he has argued, would hugely accelerate the earth's natural uptake of CO2 from the open air, at a cost of only about 10 euros per captured ton of the gas. Just 25 billion tons of olivine could therefore capture all the CO2 produced by human industry in a year for about 200 billion euros. The environmental effects of scattering so much olivine dust across the tropics are unknown, but Schuiling regards them as relatively benign.
Not everyone comes to such optimistic conclusions about the potential of mineral carbonation, however. A 2010 study in Proceedings of the National Academy of Sciences by Peter Köhler, Jens Hartmann, and Dieter A. Wolf-Gladrow of the Alfred Wegener Institute for Polar and Marine Research and the University of Hamburg also took a preliminary look at the potential of artificially expedited weathering of olivine to offset CO2 emissions and global warming. They considered the possibility of distributing massive amounts of olivine as a finely ground power over land areas in the humid tropics. They then calculated how much and how quickly the material might remove CO2 from the air and what effect that might have according to climate models.
The results were encouraging in many ways, but might fall short of the expectations of anyone who wanted to see mineral carbonation as a panacea. In short, Köhler and his colleagues determined that the olivine could help to negate higher levels of CO2 and warming if industrial emitters of CO2 leveled off -- but it could not prevent significant, potentially dangerous warming if CO2 production continues apace.
They calculated that olivine distributed in this way might take up between one billion and five billion grams of CO2 per year. In their model, if aggressive CO2 released continued, then by 2100 the global temperature might rise by about 4.9 degrees Celsius; a more optimistic scenario of curtailed CO2 release would hold the warming to about 2.5 °C -- both in excess of the 2 °C increase that most climate scientists regard as the threshold for potentially dangerous changes.
If the rate of CO2 uptake was small, the scientists found, then under either scenario, the olivine technique would shave only about 0.2 °C off the projected increase in global temperatures by 2100. If the rate of CO2 uptake was high, under the optimistic emissions scenario the olivine could cut half a degree off the increase -- enough to keep warming under the 2 °C threshold. But if emissions continued to rise aggressively, then the temperature would rise by more than 3 degrees even with the higher rates of CO2 removal.
In the discussion section of their paper, Köhler and his colleagues note:
[T]he amount of olivine necessary for these applications is huge: It lies in the range of present-day global coal production. Compared to other techniques olivine dissolution is very effective, rated as relatively safe, and moderately expensive.
They also observe that "ecosystem assessments for the expected impacts of the alkalinity rise are necessary before considering its implementation."
None of this work counts as the last word on the potential of mineral carbonation to fight climate change. Further studies may vindicate it as still more effective than Köhler and company projected, or they may show it to be more limited. Mineral carbonation might well eventually become an essential adjunct to other ways of fighting climate change. Yet it will still be costly and require considerable political will to deploy it, and even then, it probably cannot eliminate the need to curb CO2 emissions unless we are willing to risk more than a 2 °C increase in global temperatures.
Update (added 1/25/12): P. L. de Boer brings to my attention a paper that he and Schuiling very recently published in Earth System Dynamics Discussion on the rate of olivine weathering. They report that, based on their experiments, olivine particles in nature can weather much faster than has generally been recognized because of physical abrasion and biological processes that strip the passivating silica layer from their surfaces. By their calculations, spreading roughly 7 cubic kilometers worth of olivine (about 23 billion tons) annually into some of the world's most energetic shelf seas could compensate for a year's current global industrial CO2 emissions while also counteracting ocean acidification.
They write:
Olivine and other (ultra)mafic minerals in high-energy shallow-marine environments weather fast. Their application can make a significant contribution in the fight against climate change. The counteracting effect on ocean acidification is immediate. Large scale spreading of olivine in shelf seas with adequate tidal currents and wave action can be started any moment. The scale on which olivine has to be spread, asks for 15 a major expansion of olivine mining, a quintupling of the ores and industrial minerals mining industry, that is an amount more or less equal to the amount of construction minerals mined annually. This requires less effort and investments than those for the recovery of fossil fuels and CCS [carbon capture and sequestration].
If true, the odds would greatly improve that mineral carbonation could provide a technological end run around many of the usual obstacles to climate progress. Concerns about the geoengineering involved would still need to be addressed put to rest, though, and humanity would still need to muster the will to deploy this solution at scale to make it work. Still, it's encouraging. We'll see what comes of it.
•
Image: North on Highway 160, Arizona. (Credit: Alex E. Proimos, via Flickr)
This post was originally published on Smartplanet.com