What is the limit to Moore's Law?
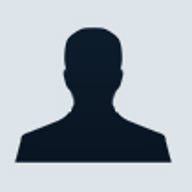
For the past three decades, Moore's Law has remained an accurate predictor of silicon device design progress.
Many people, including Gordon Moore, have unsuccessfully predicted the end of Moore's law. In 1993, as Intel began to produce 0.50-micron (1 micron is about one-thousandth the width of a human hair) devices in volume, Moore presented a keynote at a technical conference titled, "No Exponential Lasts Forever." He predicted that Moore's Law would run into serious technical and economic barriers at the 0.25-micron process generation.
We are pleased to confirm that even this prediction has been proven wrong.
Moore further elaborated on a number of technical issues. He was especially concerned about lithography and gate oxide. As gate oxide becomes thinner and thinner at every new generation of technology, he predicted that continuing to produce processors by conventional methods would result in unacceptable leakage current levels at the 0.25-micron generation. Even if technical solutions could be found, the production cost would become prohibitive.
Leak-free
The Intel research-and-development teams continued to take on the challenge of device scaling. Within four years of Gordon Moore's prediction of the end of Moore's Law, Intel put 0.25-micron devices into high volume production.
The transistor leakage current was well within acceptable limits and the performance of the 0.25-micron devices was about four times that of the 0.50-micron devices. The production cost was a small fraction of the cost of the 0.50-micron devices, thanks to improvements in lithography productivity.
In December 2000, Intel researchers presented a paper on 30-nanometer gate length (0.03 microns) transistors that demonstrated record CMOS performance. These transistors were nearly 10 times smaller than the limit Gordon forecasted in 1993.
Transistors are the engines that drive silicon device performance. In our 30-nanometer paper, we demonstrated individual CMOS transistors that have speeds faster than 1,200GHz. More impressively, these transistors demonstrated this high performance at operating voltages 35 percent lower than the 1.3-volt standard of today. We believe that these transistors will enable microprocessors with more than 400 million transistors to run at 10GHz clock speeds with the lowest possible energy consumption and heating.
Intel's 30=nanometer transistors contain features that are atomically thin. The silicon-dioxide gate was shrunk to an incredibly thin 0.8-nanometer dimension. This corresponds to an average thickness of only three atomic layers for the most critical device structure.
Intel researchers have already demonstrated good results for a new class of materials called high K gate dielectrics to replace silicon dioxide. These new materials yield the same electrical performance as silicon dioxide but have a thousand times less electrical leakage.
Only wafer-thin
The ability to print ever-smaller lines on silicon wafers (lithography) is the other driving force for Moore's Law.
One fundamental physical law is that the resolution of a lens is directly related to the wavelength of the exposure light. That is, we need shorter wavelengths of light to print smaller lines. Intel researchers are demonstrating two new lithographic techniques. The first is to reduce the deep ultraviolet light exposure wavelength by 19 percent, from 193 nanometers to 157 nanometers.
This light does not transmit through oxygen or glass, and we need to create nitrogen-purged environments and new materials to be compatible with the shorter wavelengths. The new 157nm wavelength lithography should allow us to reliably print 50 nanometer features by the middle of the decade.
The more dramatic lithography breakthrough is called extreme ultraviolet, or EUV. These tools will use an incredibly small wavelength of light (13.4 nanometers), which is 93 percent shorter than the most advanced production tools.
This light does not travel through the atmosphere or through lenses without being absorbed. It means that we had to invent vacuum systems and new types of mirrors to make these lithography tools possible. The EUV LLC, whose members include Intel, plus AMD, Infineon, Micron and Motorola, plans to demonstrate the first exposures on this revolutionary technology April 11. We expect to be able to reliably print lines less than 30 nanometers wide in the second half of the decade.
Microprocessors built based on 30-nanometer transistors will enable computing applications not possible today. A 10GHz processor could power a universal translator -- similar to a device used on Star Trek. For example, an American tourist shopping at a market in Paris could speak English into a walkie/talkie-like device. The universal translator device would then automatically translate the tourist's question and audibly relay it in French to the shop owner.
Microprocessor power will also enable your computer to remember and search for images similar to the way the human mind works. For example, imagine while you are browsing the Internet that you see a photo of a celebrity wearing a really nice sweater. You could click on the sweater and tell the computer "Find me a sweater that looks like that". It could then quickly scan Web sites and find likely matches to this image. This is similar to the way that people can quickly scan through a catalogue today and find the objects that look like the image they are trying to match.
Where does it stop?
Every exponential-growth law does come to an end. So what is the future of silicon devices as we scale dimensions down to atomic levels?
A recent study by the University of California at Berkeley surveyed all the competing technologies that could potentially replace silicon.
First, we need to recognize that most biological elements, such as cells, are much bigger than silicon devices and offer no density advantage. On a smaller scale, some organic compounds could have a long-term advantage in memory density over silicon and perhaps could enable a "self-assembly" manufacturing process. However, this density advantage is negated by the lack of power to drive high-speed communications. It appears likely that new technologies such as organic memory, optical communications, and micro-mechanical devices will have a silicon engine at the core. We anticipate that the end of the decade will bring the first applications of the marriage of organic materials and silicon devices.
Gordon's Moore's prediction is right: Moore's Law in a physical sense will end. However, the strength of our industry is that engineers and scientists continue to invent clever ways to push out the date. The end of scaling doesn't mean an end to innovation, and as long as innovation is a cornerstone of our industry, Moore's Law will never truly disappear.
Dr. Gerald Marcyk is director of components research at Intel's Hillsboro, Ore, facility.