Taking STM images 100 times faster
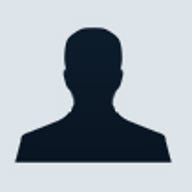
Very few of you have used a scanning tunneling microscope (STM), an essential tool to study nanoscience. And you might think that it's as easy to take a picture of an atom with an STM as it is to take a shot with your digital camera. In fact, the imaging of individual atoms with a STM is awfully slow. But now, U.S. researchers had a very simple idea to accelerate this process. By adding a simple radio transmitter, they are able to make atomic-level microscopy at least 100 times faster. A typical STM has currently a sampling rate of about one kilohertz. This new radio-frequency scanning tunnelling microscopy can operate a thousand times faster. Very bright idea!
This project has been led by Keith Schwab, an associate professor of physics at Cornell, with the help of the members of his research group and some colleagues at Boston University. On the left is a photo from Keith Schwab with a STM (Credit: Cornell University).
Before going further, why is the process of taking images with a STM so slow? "The limiting factor is not in the signal itself -- it's in the basic electronics involved in analyzing it. A theoretical STM could collect data as fast as electrons can tunnel -- at a rate of one gigahertz, or 1 billion cycles per second of bandwidth. But a typical STM is slowed down by the capacitance, or energy storage, in the cables that make up its readout circuitry -- to about one kilohertz (1,000 cycles per second) or less."
In the last 20 years, many researchers have tried to improve the situation, without success. But Schwab found a very simple solution. "By adding an external source of radio frequency (RF) waves and sending a wave into the STM through a simple network, the researchers showed that it's possible to detect the resistance at the tunneling junction -- and hence the distance between the probe and sample surface -- based on the characteristics of the wave that reflects back to the source. The technique, called reflectometry, uses the standard cables as paths for high-frequency waves, which aren't slowed down by the cables' capacitance."
And what are the results he obtained? "There are six orders of magnitude between the fundamental limit in frequency and where people are operating," said Schwab. With the RF adaptation, speeds increase by a factor of between 100 and 1,000. "Our hope is that we can produce more or less video images, as opposed to a scan that takes forever."
This research work has been published by Nature under the name "Radio-frequency scanning tunnelling microscopy" (Volume 450, Number 7166, Pages 85-89, November 1, 2007). Here is the editor's summary, "STM now on radio." "The scanning tunnelling microscope (STM) is one of the most useful tools in nanoscience. But a severe limitation of the technique is its time resolution. This is determined not by the fundamental physics of tunnelling, but by the limited high-frequency response of the conventional tunnel current read-out circuitry. The radio-frequency STM uses a specially designed radio-frequency measurement circuit to avoid these measurement bandwidth limitations and work reported in this issue shows that the 'RF-STM' can improve on time resolution by a factor of 100 compared to a state-of-the-art STM. Experimental demonstrations of the new fast-imaging instrument illustrate its suitability for three potential applications — fast surface topography, thermometry at the nanometre scale and nanomechanical displacement sensing."
And here are two links to the abstract and to the full paper (PDF format, 5 pages, 376 KB). Here is a short excerpt from the abstract. "A severe limitation in scanning tunnelling microscopy is the low temporal resolution, originating from the diminished high-frequency response of the tunnel current readout circuitry. Here we overcome this limitation by measuring the reflection from a resonant inductor–capacitor circuit in which the tunnel junction is embedded, and demonstrate electronic bandwidths as high as 10 MHz. This 100-fold bandwidth improvement on the state of the art translates into fast surface topography as well as delicate measurements in mesoscopic electronics and mechanics."
Sources: Lauren Gold, Cornell Chronicle Online, October 31, 2007; and various websites
You'll find related stories by following the links below.