Exploding batteries: A serious charge
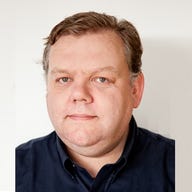
Exploding lithium-ion batteries have sunk Samsung's flagship Galaxy Note 7 and damaged the company's bottom line.
Phone companies often talk about bang-per-buck, but rarely mean it literally. Explosive devices are unpopular outside battle zones, so when the Galaxy Note 7, Samsung's now-discontinued flagship smartphone, started to combust in appreciable numbers, something was obviously wrong. The culprit proved to be the lithium ion (Li-ion) battery -- unsurprisingly, as that component stores the energy needed to run all the advanced electronics, high-speed radio links and large, bright screen that characterise today's top-tier handsets.
It's at this point that physics, marketing and expectation come into conflict. Users expect more brightness, more processing power and more features with every phone generation, but are not prepared to trade off phone slimness or life between charges. The Galaxy Note 7 battery stores 3,500 milliamp-hours (mAh), which at 3.7 volts checks in at around 13 watt-hours (Wh) -- all in a very slim, light package. That might not sound much, but Li-ion batteries are very good at accepting and delivering power quickly, which is what makes them the default technology for electric cars such as those made by Tesla.
It's not such a good idea in the trouser department. If all the power in a fully-charged Note 7 battery was used up in ten seconds, it would clock in at 4 kilowatts, which is the equivalent of several hundred bunsen burners in your pocket. As Li-ion batteries contain pressurised, combustible materials, the result of an internal short circuit -- when the battery will indeed do its best to produce as much energy as quickly as possible -- are spectacular. This is probably what happened in the Galaxy Note 7, due to distortions produced in the battery by the case, although we await the final report.
This, of course, is unacceptable, even if the fault occurs one time in a million -- the sort of level that in any other component would be hard to detect even by statistical' analysis. With tens of millions of some units in the field, and social media able to fan the flames, it doesn't take much to kill a product.
Li-ion batteries were considered too dangerous for general consumer use when first invented in the 1970s. However, lithium is a superb material for batteries -- it's the lightest metal, with excellent electronic properties -- and after considerable work on safe construction and use, Sony produced the first consumer-grade batteries in 1991. All early Li-ion batteries used a liquid electrolyte -- the medium that allows ions to move between electrodes and thus electricity to flow. In 1994, the first economic polymer electrolyte matrix was announced, which is much safer, better able to be formed in different shapes, and increases energy density without increasing volume. Even so, it took until 2009 before the first lithium-polymer (Li-poly) batteries appeared in consumer products.
Given that lithium-based batteries have attracted by far the most investment and commercial interest in the mobile power market, it's clear that significant improvements in battery technology take a long time to reach production. With Li-ion technology still improving at around five percent a year, alternative approaches face a fearsome challenge. But as Samsung has learned to its cost, today's batteries are far from perfect.
Here are the frontrunners to answer this burning question.
Magnesium
Magnesium-based rechargeable batteries are getting a lot of interest. Magnesium has twice as many electrons available for electrochemistry as lithium, and has getting on for twice the theoretical energy density. It's also safer and doesn't degrade as much during the charge-discharge cycle -- lithium has a tendency to grow dendrites, sharp spikes that can disrupt a battery's working, which magnesium does not. This simplifies the internal construction of the battery, making it potentially cheaper and smaller, as well as giving the cells a longer service life. Plus, magnesium isn't as reactive in air as lithium, making it safer.
Interaction between a magnesium anode and conventional electrolytes can result in the formation of an impermeable 'passivation' layer. The similar 'solid electrolyte interface' (SEI) in lithium batteries allows for lithium ion diffusion.
Finding electrolytes that work well has been challenging. Magnesium can form 'passivation' layers, a chemical coating around the anode that drastically reduces battery performance, and electrolytes that combat this while remaining stable and not being corrosive have been hard to find. Cathode chemistry has also been problematic, with early candidates suffering from permutations of poor low-temperature performance, much lower voltages than lithium, or lifetime issues.
However, in early 2016, Toyota published a paper describing a new kind of electrolyte based on research done into hydrogen storage. Although magnesium batteries remain some way from a plausible path to production, they are a promising bet.
Graphene
Graphene is the famous single-atom-thick hexagonal sheet state of carbon, created in 2004 at the University of Manchester. Among its many remarkable properties, graphene's high conductivity and strength combined with its extreme thinness has resulted in it being used to enhance electrode structures and improve efficiency in existing battery technologies -- including Li-ion, which already uses carbon in the electrodes to stabilise the metal's behaviour.
The most exciting development may be in graphene super- or ultra-capacitors. Capacitors store charge, like batteries, but in electrostatic rather than ionic potential: charge is built up on two conductive sheets held apart by a thin non-conductive insulator. Capacitors can receive and return charge very much faster than chemical batteries, but with a vastly lower energy density; they also have a constantly decreasing voltage while discharging.
The structure of an ultracapacitor. Graphene can be used to boost the energy density, which is typically orders of magnitude lower than for a chemical battery.
With graphene, however, the energy density can in theory be hugely increased as the surface area of a tightly rolled electrode structure can be very large indeed -- some manufacturers claim more than five times that of Li-ion is practicable. They can also be charged with lots of power very quickly -- 30 times faster is one claim -- as the highly conductive electrode does not lose much energy in heating even for large currents, and no physical movement of electrolyte constituents is needed. Finally, they don't wear out nearly as fast as electrochemical cells, although there are many problems in producing large-scale atomic-level devices of high reliability. So far, the technology remains in the labs.
As the history of practical graphene production and engineering is extremely short, it's likely that the first practical applications will be in electric vehicles, where the market is currently being restricted by charging times and range. Both of these metrics could be greatly boosted by large graphene power stores, be they electrochemical or electrostatic.
Novel lithium chemistry
Two rather different lithium-based cell types are also worth watching. The first, lithium-sulphur (Li-S), replaces the lithium cathode of an ordinary Li-ion battery with a carbon-sulphur combination and a lithium sulphide electrolyte. This produces a passivation layer on the lithium anode, but rather than impede efficiency it stabilises the anode without needing further protective structures. This increases energy density -- demonstration units have delivered more than twice Li-ion's capability, with much more promised. The downsides have been a tendency for unseemly combustion, unstable electrolytes, slow charging and a service life of a few hundred charges.
Li-S batteries have been around for a while for specialist applications, without mass production taking off -- promised significant milestones have been regularly missed. Regular improvements are reported and the British company Oxis Energy is conducting live EU-sponsored tests with a Seat Leon electric vehicle. Mobile phone users may have to wait a little longer, as Sony is predicting production for 2020 with a 40 percent advantage over Li-ion. In the meantime, the technology even has its own conference at the Royal Institution, so the outlook is good.
Another lithium system that's often mentioned is lithium-air (Li-Air), which uses atmospheric oxygen combining with lithium ions. This has the overwhelming advantage that, in theory, it can exhibit the highest possible energy density (close to that of petrol and over fifty times better than Li-ion), meaning either very small size or very high performance. It also has overwhelming disadvantages of very poor efficiency, poor charge/discharge times, a high chance of contamination and a higher chance of violent self-destruction, as combining oxygen and lithium is exactly the same chemistry behind burning smartphones.
For these reasons, Li-Air languished on the lab shelf (behind blast doors) until about five years ago. Although recent developments have been encouraging, with advances on all fronts, and you'll read a lot about Li-Air in the future, don't expect to see it in action before the middle of the next decade, at best.
Many other metal-air batteries are either in development or even in use -- zinc-air non-rechargeable batteries are among the smallest long-life cells, used in hearing aids and the like, and you'll see iron-air and aluminium-air presented as potential Li-ion replacements. All await further research into the significant practical problems that currently exclude them from the mass market.
And the rest
The combination of environmental concerns and ubiquitous mobile technology has spurred a vast amount of research in many other exotic battery technologies, as well as a plethora of biological, nanotech, fuel cells and wireless power propositions. You can make batteries out of hundreds of combinations of materials, and someone's trying to sell most of them.
In general, these need to be judged carefully: many are barely more than persuasive marketing designed to extract research funding or state investment; others are borne aloft on the well-meaning hopes of their creators, who are (often `misguidedly) optimistic that their particular solution will be spared the worst of the typically decades-long development path to safe, economical, competitive products.
Look for peer-reviewed scientific papers. Look for many companies working on the same or similar ideas. Look askew at glossy videos -- especially where no solid technical information is available. Track record and solid investment history are the best guide, but are still no guarantee.
For the foreseeable future, then, we will be using Li-ion batteries of one sort or another in our mobile devices -- even if they retain the potential to bite back once in awhile. Li-ion and its offspring still have much potential for improvement, and the undivided attention of some very experienced and well-funded outfits. The next generation of Samsung phones will still be powered by Li-ion -- aided, you can be sure, by better engineering where it matters.
Read more